Equine influenza virus, equine herpesviruses 1 and 4 (EHV-1 and EHV-4) and Streptococcus equi subspecies equi (S. equi) are endemic in the UK and carry significant clinical, economical and welfare consequences for all involved. They are not currently notifiable diseases in the UK, but the high morbidity and outbreak potential of these pathogens pose a great challenge to veterinarians, and early recognition followed by prompt treatment and control are required. Good biosecurity and vaccinations are the most effective ways to prevent outbreaks.
Equine influenza virus
Equine influenza is endemic in the UK and is a major cause of respiratory disease worldwide. The disease causes substantial socio-economic losses; it halted racing in Great Britain in 2019, and an Australian outbreak in 2007 cost around $1 billion AUD (Equine Infectious Disease Surveillance, 2024).
Influenza A is part of the Orthomyxoviridae family and two subtypes, H7N7 and H3N8, are pathogenic in horses. Subtypes of the virus are named based on the antigenic variants of the surface proteins haemagglutinin (H), responsible for viral attachment to the host cells, and neuraminidase (N), which plays a key role in the release of the replicated virus (Singh et al, 2018). H3N8 is the only variant currently causing disease (Myers and Wilson, 2006). In the 1980s, genetic evolution of H3N8 resulted in the formation of the Eurasian and American lineages. The American lineage diverged into three subtypes: Kentucky, South American and Florida, which further diverged into clade 1 and clade 2 (Figure 1). Clade 1 predominates in America, but has been found in Europe, Australia, Africa and Asia, while clade 2 has been isolated in Europe, Africa and Asia. Because the H7N7 and the Eurasian lineage have not been isolated in horses since the late 1970s and 2005, respectively, the advice of the Office International des Epizooties Expert Panel for equine influenza is not to include them in equine influenza vaccines, but strains representatives of both clade 1 and clade 2 should be included in killed vaccines. From 2016, all isolated equine influenza viruses were from the Florida lineage, with clade 1 identified in the US and clade 2 in Europe (World Organisation for Animal Health, 2022).
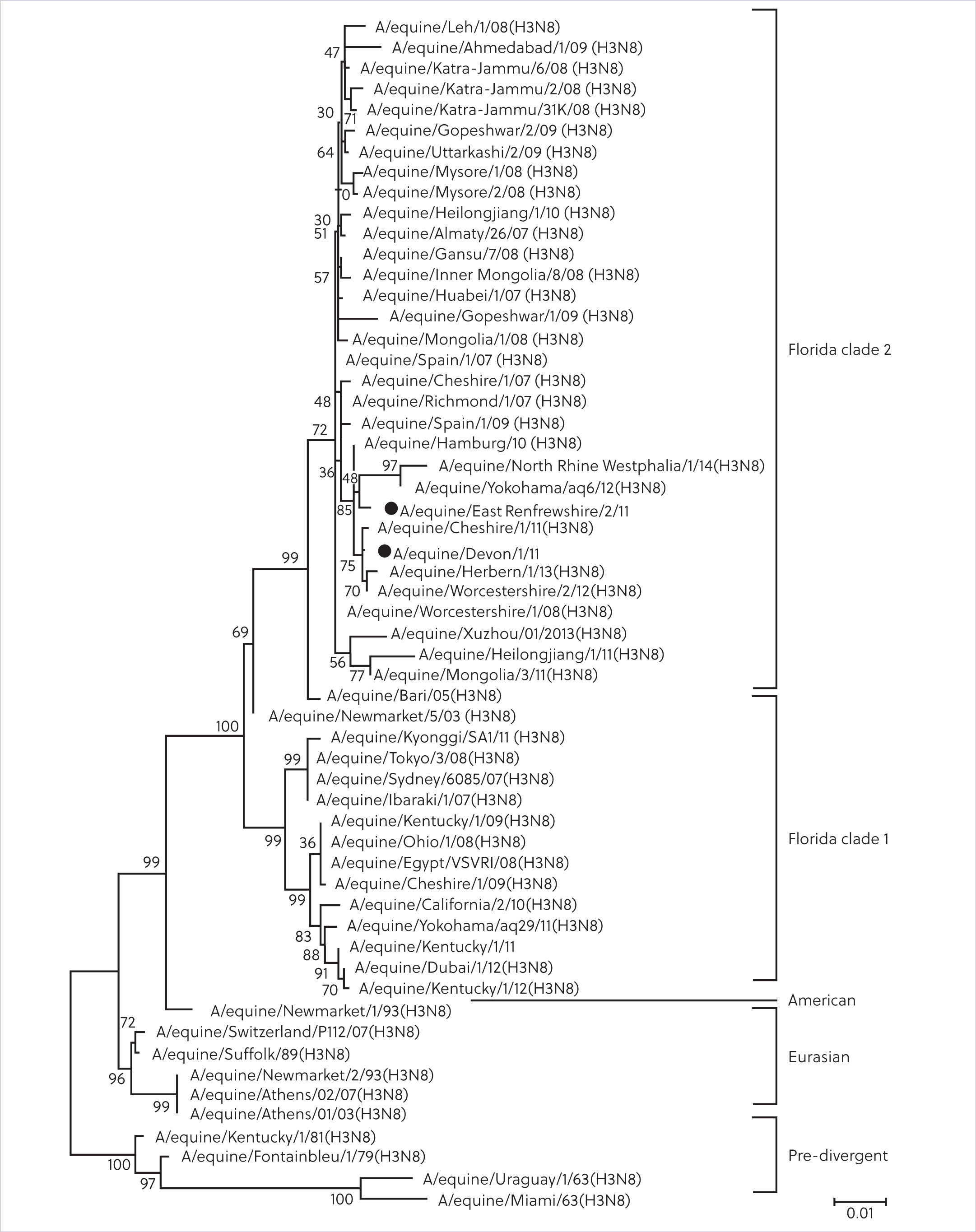
The virus is transmitted by aerosol, direct contact with infected animals and indirect contact (Cullinane and Newton, 2013). Humidity of less than 60% and wind speeds greater than 30 km/hour are associated with an increased risk of spread, and in optimal conditions it has the potential to spread up to 1.5 km (Firestone et al, 2012). Initial clinical signs include fever, anorexia, lethargy and dry cough (Myers and Wilson, 2006). Vaccinated horses can still become infected, but clinical signs are usually milder and of shorter duration, making it more difficult for owners and veterinarians to recognise (Box 1). The incubation period lasts between 1 and 3 days, with shedding starting as soon as 24 hours post infection. In naïve animals, shedding can last for up to 10 days (Daly et al, 2004).
Equine influenza vaccination summary
Vaccination status should be considered when interpreting the clinical severity of disease. Influenza should be suspected in horses displaying relevant clinical signs in a group scenario with a history of recent travel or potential recent exposure (eg recent competitions or new horse on the yard). Gatherings, such as international competition events and global transportation of horses, have been associated with numerous outbreaks through the introduction of novel strains into previously unexposed horse populations (Myers and Wilson, 2006).
Affected horses should be sampled as soon as the clinical signs start, which is typically 2–5 days after exposure and correlates to maximal peak of viral load (Mumford et al, 1990). While virus isolation is labour intensive and time consuming, it remains crucial for surveillance and can inform vaccine strain selection. Diagnostic techniques and sampling are summarised in Table 1.
Test | Advantages | Disadvantages | |
---|---|---|---|
Agent detection (nasopharyngeal swab) | Virus isolation | Highly specific |
Slow, can take up to a few weeks |
Reverse transcriptase polymerase chain reaction | Rapid (2 hours) |
Chances of false positive because of high sensitivity |
|
Antibody detection* (paired serology: serum samples) | Antigen capture enzyme-linked immunosorbent assay | Quick to run |
Requires two serum samples |
Haemagglutination inhibition | Quick and easy |
Only detects antibodies to the viral subtype being tested for | |
Single radial haemolysis | Quantitative results |
Requires trained personnel |
The World Organisation for Animal Health has an expert surveillance panel that meets regularly to discuss equine influenza vaccine composition and provide recommendations. The most recent recommendations were given in July 2022 and remain unchanged since 2010, stating that influenza vaccines should contain both clade 1 and clade 2 of the Florida sublineages (World Organisation for Animal Health, 2022). ProteqFlu (Boehringer Ingelheim) is currently the only licenced product containing both clades in the UK.
Herd immunity for equine influenza requires 70% of the UK horse population to be vaccinated for an outbreak to be prevented, with this proportion rising to 95% if a change in strain occurs (Park et al, 2009). While there are challenges to accurate quantification of the proportion of vaccinated horses, currently it is estimated to be around 30% in the UK, which could pose a significant threat for future outbreaks (Whitlock et al, 2023).
The primary course consists of two vaccinations (V1 and V2) 4–6 weeks apart, followed by a third (V3), 5–6 months following V2. Further boosters are then administered every 6–12 months. Antibody titres can be measured post vaccination by single radial haemolysis, though this is rarely performed in clinical practice. In research studies, single radial haemolysis levels have been shown to correlate with protection against infection, with a reduction in both clinical signs and viral shedding (Paillot et al, 2013a). Importantly, antibody titres have been shown to decrease significantly between V2 and V3, leaving some horses susceptible to influenza infection (Paillot et al, 2013b). This period of vulnerability is known as an immunity gap. During the immunity gap, vaccinated horses are susceptible to influenza and might develop mild clinical signs or asymptomatic shedding, posing a significant risk to unvaccinated naïve horses (Murcia et al, 2013). Considering this, the British Horseracing Authority and International Equestrian Federation have recently shortened the vaccination interval (Table 2). Under the new British Horseracing Authority rules, the primary course (V1–V2) must be given 21–60 days apart, with the third vaccination (V3) 120–180 days after the second. Horses should receive a booster vaccination no more than 6 months apart. Horses failing to comply with the 6-monthly booster vaccination will need to restart the course (British Horseracing Authority, 2022). Horses competing under International Equestrian Federation regulation require a second vaccination 21–92 days after the first, V3 within 215 days of V2 and are required to have been vaccinated within 6 months and 21 days before a competition. They must not have been vaccinated within 7 days before competing or arriving at a competition venue (International Equestrian Federation, 2024).
Historic intervals | British Horseracing Authority | International Equestrian Federation |
---|---|---|
V1–V2: 21–92 days | V1–V2: 21–60 days | V1-V2: 21–92 days |
V3: 150–215 days | V3: 120–180 days | V3: within 215 days |
Booster: no more than 1 year apart | Booster: no more than 6 months apart | Booster: within 6 months and 21 days preceding a competition |
One study showed a 100% infection rate in vaccinated horses when exposed to influenza at day 185 after V2; however, vaccinated horses had significantly milder and shorter clinical signs, a shortened period of shedding and no need for antibiotic treatment throughout the infection, compared with control non-vaccinated horses (Paillot et al, 2018). With the current influenza vaccines, it may not be possible to close this immunity gap, because a shortening of the V2–V3 interval has been shown to be counter-productive, with a detrimental effect on long-term humoral immunity, because seroconversion does not seem to occur after the fifth vaccination in an accelerated schedule (Heldens et al, 2007). However, in the event of an outbreak, a booster influenza vaccination should be considered, especially in horses where the vaccination protocol has been started recently (Paillot et al, 2017).
Equine herpesvirus
EHV is part of the Herpesviridae family. Herpesviridae are divided into three groups: alpha, beta and gamma (Box 2). Numerous types have been identified in horses, but the most clinically relevant are EHV-1, EHV-3 and EHV-4 (alpha-herpesviruses) and EHV-2 and EHV-5 (gamma-herpesviruses) (Table 3). EHV-1 and EHV-4 are the most relevant pathogens clinically and economically (Patel and Heldens, 2004). EHV-1 is typically associated with respiratory infections in young horses, but can have more serious consequences, such as late-term abortion, neonatal death and equine herpesvirus-1 myeloencephalopathy (Dunowska, 2016). EHV-4 is usually limited to the upper respiratory tract (Ma et al, 2013).
Equine herpesvirus summary
Group | Types | Clinical signs |
---|---|---|
Alpha | EHV-1 | Respiratory disease, abortions and abortions outbreaks, sudden death in foals, neurological disease and outbreaks |
EHV-3 | Coital exanthema | |
EHV-4 | Respiratory disease, abortion | |
Gamma | EHV-2 | Keratoconjunctivitis |
EHV-5 | Equine multinodular pulmonary fibrosis |
EHV is spread through inhalation of aerosols, direct contact with infected placental tissue, aborted fetuses and infected animals, and indirect contact with fomites. After inoculation, the virus colonises the respiratory epithelium, damaging the respiratory mucosa (Lunn et al, 2009). The initial clinical signs of pyrexia and serous nasal discharge can be seen at this stage. Within 24–48 hours, the virus can be detected in local lymph nodes of the respiratory tracts, where it infects monocytes and CD4+ and CD8+ T-lymphocytes. These are then released into the bloodstream, resulting in cell-associated viraemia 4–10 days post-inoculation. This cell-associated viraemia is responsible for a second phase of pyrexia (Goehring et al, 2024). At this stage, EHV-1 cell-associated viraemia carries the virus to tertiary replication sites, such as the endothelium of pregnant uterus, the central nervous system and the retina, resulting in abortion, neurological syndrome and chorio-retinal lesions respectively. Concurrently, the virus gains access to the trigeminal ganglion and establishes latency (Oladunni et al, 2019). Current latency estimates are variable depending on geographic region, management conditions and age, varying from 25.7% (Pusterla et al, 2012) to over 60% (Lunn et al, 2009). However, latency prevalence has been shown to be 100% at 70 days post infection in experimental studies (Giessler et al, 2020; Samoilowa et al, 2021), supporting the necessity for long-term in-vivo studies. There is currently no way to definitively diagnose latency in the live horse.
When EHV-1 is suspected, it is important to consider the timing of sampling and stage of disease. EHV-1 shedding in the respiratory tract can be detected by real-time quantitative polymerase chain reaction, which can be also used to determine viral load. EHV-1 can be detected on nasopharyngeal swabs from 1–2 days post infection up to 2–3 weeks, whereas cell-associated viraemia can be detected on heparinised blood samples from 5–7 days and up to 2–3 weeks post infection (European Food Safety Authority et al, 2022). Shedding may be intermittent and short-lived, so samples should be collected early in clinical cases when pyrexia first appears (Slater, 2014). Ideally, quantitative polymerase chain reaction should be performed on both nasopharyngeal swabs and heparinised blood (30–40 ml) for a higher diagnostic sensitivity. A positive polymerase chain reaction result should also be followed by viral isolation (Lunn et al, 2024) to identify the strain of the virus, provide characterisation of the viral isolate, aid in viral understanding and provide targets for novel live attenuated vaccines (Slater, 2014). In the event of an inconclusive polymerase chain reaction result, seroconversion can be confirmed with paired blood samples (acute and convalescence samples, taken 14 days apart). A 4-fold or greater rise in virus-specific antibody titre detected by complement fixation or viral neutralisation is indicative of recent infection.
Treatment is mostly symptomatic and supportive. Vaccinations are available, but do not provide adequate cell-mediated immunity, resulting in incomplete protection (Goodman et al, 2012). Two meta-analyses (Marenzoni et al, 2023; Osterrieder et al, 2024) showed that commercially available vaccines minimally reduce the incidence of clinical disease and shedding associated with infection, and there is no evidence that current vaccines can prevent naturally-occurring equine herpesvirus myeloencephalopathy (Pusterla and Hussey, 2014). Antiviral agents, such as the acyclic guanosine analogues acyclovir, ganciclovir and valganciclovir, have been studied against EHV-1. Oral acyclovir in horses results in poor bioavailability and low plasma levels (Razonable, 2011) and no effect is observed on viraemia, virus shedding or clinical outcome (Garré et al, 2009). In-vitro studies showed that effective ganciclovir and valganciclovir concentrations can be achieved in horses (Vissani et al, 2012; Carmichael et al, 2013) and the drugs now need to be tested for safety and dose efficacy to be considered as potential treatments (Vissani et al, 2016).
Control and prevention of the disease should be achieved with management procedures, such as segregation of pregnant mares from other horses, isolation and testing of new horses on the premises and separating of transiting competing horses from permanent residents. In the event of an EHV-1 outbreak, it is advised to maintain quarantine measures for 28 days after resolution of clinical signs, because viral shedding may continue for up to 21 days post infection. The virus may survive for longer than 48 hours in the environment and disinfectants, heat, detergents and lipid solvents have a virucidal effect (Saklou et al, 2021).
Strangles
Strangles is a highly contagious infection of the upper respiratory tract in horses caused by the gram-positive bacteria Streptococcus equi subspecies equi (S. equi) (Box 3).
Strangles summary
The first clinical sign of infection is fever, caused by the release of pyrogenic mitogens, followed by anorexia, bilateral mucopurulent nasal discharge, depression, lethargy and abscessation of retropharyngeal and sub-mandibular lymph nodes (Sweeney et al, 2005). Early diagnosis is valuable, but timing of testing must be considered, with different methods required at different stages of the disease. Following inoculation, S. equi attaches to the mucosal-associated lymphoid tissue of the upper airways. At this stage, polymerase chain reaction or bacterial culture of the nasal or nasopharyngeal regions may provide false negative results, as the bacteria is not present on the mucosa until 24–48 hours after onset of pyrexia (Boyle et al, 2018). Translocation to the retropharyngeal and mandibular lymph nodes occurs within few hours, attracting a large number of neutrophils. Lymphadenopathy becomes evident within 3–5 days, and abscesses mature and rupture between 7 and 14 days (Boyle et al, 2018). Depending on the location of the lymph nodes, abscesses may drain externally through the skin or into the guttural pouches, causing empyema (Judy et al, 1999). If sampling is performed before guttural pouch colonisation, guttural pouch washes will return false negative results.
Shedding begins 2–3 days after the onset of fever and persists for 2–3 weeks in most cases, but it can continue for as long as 6 weeks after the discharge has resolved (Sweeney et al, 2005). Approximately 10% of affected horses become subclinical carriers, developing chronic guttural pouch empyema or chondroids, which harbour and periodically shed S. equi for prolonged periods (Sweeney et al, 2005). These horses are called subclinical carriers and can be a source for outbreaks and persistent disease in the population (Duran and Goehring, 2021).
Quantitative polymerase chain reaction is three times more sensitive than culture and returns results rapidly (Newton et al, 2000). It can be performed on nasopharyngeal swabs or lavages, and guttural pouch lavages. Nasopharyngeal lavages are more sensitive than swabs, but guttural pouch washes remain the gold standard for detection of subclinically infected carrier animals (Boyle et al, 2018). Caution should be exhibited with early use of guttural pouch sampling as false negatives do occur before colonisation.
Bacterial culture is widely available, but specificity might be as low as 40% in the early stage of infection (Webb et al, 2013). Paired serology can be performed with a first sample in the acute stage of the disease and a second sample 10–14 days later (convalescent). Different serological enzyme-linked immunosorbent assays are available, targeting total immunoglobulin G antibodies against different S. equi surface proteins (McLinden et al, 2023). A 4-fold or greater increase in immunoglobulin G between the paired samples is indicative of recent infection. Titres of >12 800 are suggestive of purpura haemorrhagica and/or disseminated metastatic abscessation of S. equi. However, serology is of poor diagnostic value when used to screen for silent carriers, with a sensitivity of 11.1% (Durham and Kemp-Symonds, 2021). Explanations for this paradox have been hypothesised and include shielding of antigenic epitopes when S. equi is internalised in purulent material, development of immune tolerance of a latent organism, not perceived as a threat to the host or occurrence of mutations of the S. equi genome within the guttural pouches (Harris et al, 2015; Durham and Kemp-Symonds, 2021).
Live attenuated vaccines given intranasally or sub-mucosally are commercially available in the USA and New Zealand, but they have been associated with adverse reactions, clinical signs of disease and it is not possible to differentiate vaccinated from infected animals. Equilis StrepE (MSD Animal Health) was a licenced live-attenuated vaccine administered submucosally, available in Europe and the UK, but the uptake has been poor as a result of the short duration of protection (3–6 months), adverse reaction at the injection site and, again, the inability to distinguish vaccinated animals from infected on serology (Rendle et al, 2024).
In 2022, Dechra launched a novel multi-chimeric recombinant vaccine, Strangvac, which does not contain live bacteria, but recombinant proteins of S. equi (Robinson et al, 2018). The vaccine does not cross react with enzyme-linked immunosorbent assay serology or polymerase chain reaction, making it possible to differentiate infected from vaccinated animals. The primary course includes two intramuscular vaccinations performed 4 weeks apart, followed by 6-monthly vaccination (Robinson et al, 2020). Booster vaccination is recommended 3 months after the second vaccination is administered for horses at high-risk of disease (Rendle et al, 2024). Strangvac has been shown to be safe and effective in reducing the severity and duration of clinical signs in a study done in experimental naïve ponies (Robinson et al, 2020). To reduce the risk of infection, the vaccination would have the highest benefits if horses at the highest risk of exposure are vaccinated. This includes horses kept in premises with a large number of new arrivals or horses transiting, premises with large number of yearlings and foals, studs and premises with poor biosecurity. Further work is required to assess the efficacy of the vaccine in the field and safety in pregnant mares and breeding stallions, but the capability to differentiate between natural infection and vaccination, vaccine efficacy and safety are encouraging steps towards protection against strangles.
Conclusions
Respiratory infectious diseases require prompt diagnosis and isolation to prevent an outbreak. Horses should be isolated as soon as pyrexia is acknowledged, with prompt diagnostic testing performed to identify the pathogen. Timing of testing is critical, and a good understanding of the disease pathogenesis is necessary to know which test is most suitable depending on the timing of the disease. Vaccination is a critically important tool for the prevention of infection and outbreaks, though efficacy is variable depending on which pathogen is being vaccinated against. High levels of vaccine uptake are required to promote herd immunity. To reduce the risk of an outbreak, it is important to vaccinate all horses on premises with a large number of horses transiting, new arrivals, foals and young horses, and studs accepting walk-in mares. Further studies are required to improve equine influenza vaccinations, since several are licenced and effective on strains that are no longer in circulation. Considerable knowledge gaps remain on the understanding of EHV. The novel vaccine for strangles, Strangvac, is an exciting new tool, but further studies are needed to assess and maximise its efficacy in the field. There is no replacement for quarantine of new horses entering a yard, but facilities for this are scarcely available, so continuous research for more effective vaccines and owner uptake and biosecurity are crucial to decrease the spread of infectious diseases and the number of outbreaks. At this stage, the main strategies for reduction of outbreaks of equine respiratory disease remain early diagnosis and implementation of appropriate biosecurity measures.